Organic Light-Emitting Diodes
OLEDs are built from one or more layers of organic and hybrid material (either small molecules or polymers) sandwiched between two electrodes (e.g., indium tin oxide), all on a plastic or another type of substrate. Unlike other display technologies, which require a backlight in order for the display to show, OLEDs generate their own light via electroluminescence and therefore they do not require backlights. They require less power and are more energy-efficient than backlight-dependent display technology.
OLEDs are already widely commercialized in many Samsung and other smartphone models. The Samsung Galaxy line of OLED-based smartphones occupies a significant portion of the global smartphone market. Additionally, Samsung and LG Electronics have both announced forthcoming launches of large-screen OLED TVs. The new TVs are expected to not only be more spectacular than today’s TV technology, with respect to crisper colours and sharper contrasts, but also lighter, thinner, and more energy-efficient.
A key challenge today in the field of OLEDs is the need to develop high efficiency and long lifetime, blue OLEDs. Blue light can be transformed into red and green by internal or external colour conversion, which would make the fabrication of red-green-blue (RGB) OLEDs far simpler and dramatically increase manufacturing economies-of-scale. To produce the electroactive materials required by OLEDs, conjugated organic molecules are often used. A key attraction of these organic compounds is the possibility to tailor their photophysical properties: fluorescence, charge pair production, exciton formation by charge injection, and photo-excitation. Also, these organic materials exhibit high electroluminescence efficiency.
For optimal operation of OLEDs – based on the conjugated compounds – a balanced distribution of injected charge carriers—positive (holes) and negative (electrons) – needs to be maintained. This ensures the effective recombination of these individual charges and as a result, improves the OLED efficiency significantly.
However, a large energy gap, high ionization potential, or low electron affinity often leads to the disruption of the desired equilibrium. The control of these characteristics is achieved by influencing the effective conjugation length as well as the introduction of electron-donating or electron-accepting substituents to the chromophore. A significant change in the structure of conjugated compounds also leads to changes in the specific electronic and photophysical properties or to changes in the mobility of charge carriers.
Consequently, it is anticipated the ERA Chair Team will investigate the many parameters influencing OLED emission and how changing one working and/or processing parameter may influence another one. OLED devices are typically divided into fluorescence and phosphorescence devices. Fluorescence is a fast relaxation process, where the excited molecule in the excited singlet state returns to the ground state by emitting energy in the form of photons.
To further increase device efficiency, phosphorescence emitters were developed, which allowed obtaining almost 100% internal quantum efficiency by harvesting both triplet and singlet excited states (see figure below). However, the main problem with phosphorescence emitters is that they are based on heavy metal complexes: in many cases, very expensive and scarce iridium complexes are used. To remove the heavy metal complexes from OLED emitters, while retaining high efficiency, there are two approaches: the first is to employ room temperature phosphorescence (RTP) emission and the second is to use delayed fluorescence (DF) (see figure below).
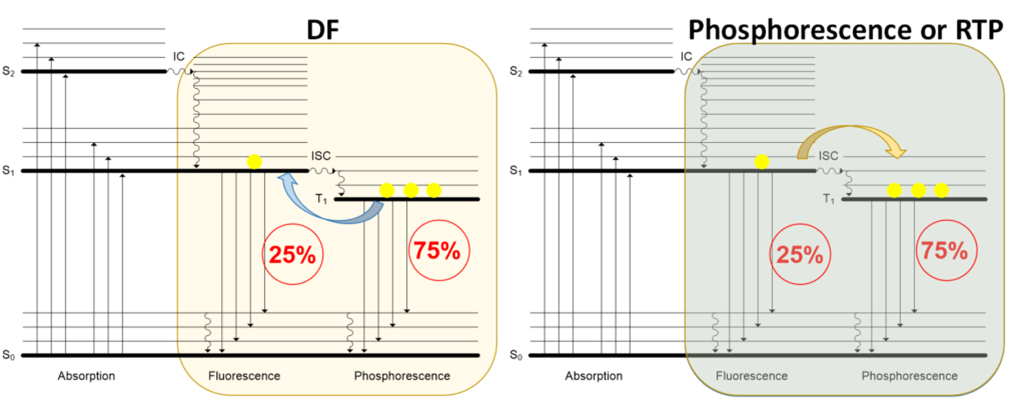
Recently, information has been published about the RTP effect in small molecular organic compounds, but all attempts so far at creating a stable, efficient OLED device have failed. Although there are examples of RTP in OLEDs, the contribution is very low. DF also allows obtaining almost 100% internal quantum efficiency from a triplet exciton, without using an iridium phosphorescent dopant. Here, a molecule excited to the triplet state returns to the singlet excited state and then relaxes to the ground state by emitting photons.
A key challenge the ERA Chair Team is expected to examine will be ways to create efficient warm white OLED devices based on non-heavy metal emitters, by mixing three RGB emitters or by employing both TADF and RTP processes. The TADF and RTP processes seem to be opposite ones but, theoretically, it is possible to connect them and receive an emitter that will have both effects depending on the working temperature. Phosphorescence is usually visible at low temperature and TADF at high (room) temperature. There are several ways to create efficient RTP emitters. In the RTP one can observe phosphorescence at room temperature, but for that one has to decrease molecular vibration by attaching big steric hindrance on the donor. To have an efficient TADF emitter, the vibration between the donor and the acceptor part of the molecule are necessary. Thus, it means that the parameter, which can increase the fluorescence TADF/RTP contribution, is a singlet-triplet gap; if the gap occurs on the edge of TADF/TTA (Triplet-Triplet Annihilation) process, it will be easier to enhance the RTP emission.
By connecting the above information, it should be possible to create a molecule that can have both RTP and TADF at room temperature. The main problem is that the colour of emission may be temperature sensitive, but this would also open a new area of application for such OLED emitters.